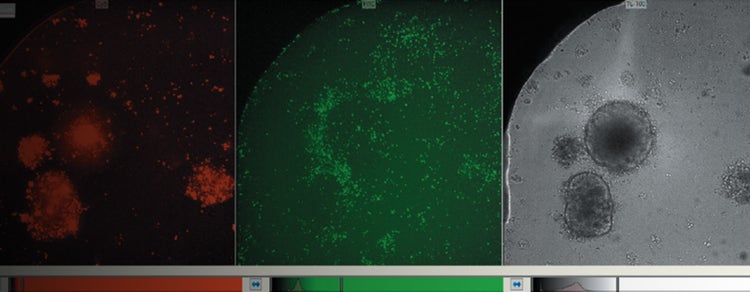
T-cells and 3D cell models for immunotherapy
Using the power of T-cells with organoids and spheroids for cancer therapy research
What are T-cells?
T-cells – also known as T lymphocytes – are infection-fighting white blood cells found in the human body. Their primary role is to help determine an immune system’s response to foreign substances, called antigens. They are complemented by B-cells – another main lymphocyte type. Combined, millions of T- and B-cells circulate throughout the body, each complete with unique receptors that enable a response to virtually any antigen.
T-cells develop from stem cells in the bone marrow and make their way to a small gland in the lymphatic system called the thymus, where they proliferate and mature. This is why they are called T-cells (thymus-derived cells). Here, they transform into specialized subtypes like, helper, regulatory, cytotoxic, and memory. When ready, the T-cells are distributed to peripheral tissues or circulate in the blood and lymphatic system to perform their jobs upon encountering an appropriate antigen. Helper T-cells, for example, will release chemical messengers called cytokines that promote the transformation of B-cells into plasma cells, which are antibody producers. Regulatory T-cells will modulate immune responses to prevent overactivity, while cytotoxic T-cells target and destroy infected or cancerous cells.
The 3D Custom Module Editor interface with mitotracker (left), T-cell (middle) and TL channel (right).
Applications of T-cell and organoid co-cultures for cancer therapy research
Immunotherapy, which leverages the immune system to specifically target cancer cells, is gaining traction as an additional form of cancer treatment. These therapies include CAR T-cells (Chimeric Antigen Receptor engineered T-cells), tumor-infiltrating lymphocytes (TIL), and other genetically modified T-cells.
While effective in treating various non-solid – or hematological – cancers, it has limited success in patients with solid tumors, which account for most cancers. One reason for the high failure rate is the immunosuppressive tumor microenvironment (TME).
Enhancing T-cell recruitment and cytotoxic activity is crucial for successful cancer immunotherapy. In preclinical scientific research, 3D cell models offer advantages over 2D monolayer cultures by better mimicking the TME’s physical and chemical conditions.
For example, patient-derived organoids (PDOs) are superior to traditional 2D monolayer cultures because they better replicate the physical and chemical cues of the TME. Studies indicate that PDOs respond to drugs similarly to original tumors, highlighting their potential to improve therapeutic outcomes. PDOs also offer advantages such as inherent heterogeneity, long-term stability, suitability for high-throughput screening, and a better ability to capture tumor characteristics, making them a superior preclinical model system.
Workflow example: T-cell-induced morphological changes of CRC organoids
T-cell research is a multifaceted and dynamic field that can encompass a broad range of studies depending on the specific goals of the researchers. The primary aim behind any T-cell research project often dictates the experimental design, including the choice of models and workflows.
Here is one workflow example designed to analyze T-cell-induced morphological changes of colorectal cancer (CRC) organoids:
Steps 1-5) Culture organoid models – This workflow begins with growing bioreactor-expanded patient-derived colorectal cancer (CRC) organoids, though a widely-used cell line can instead be used to generate models as an alternative to off-the-shelf PDOs. Cells are then seeded into plates. While not shown, automation can be leveraged throughout this process to increase walk-away time.
Steps 6-7) Ready T-cells for co-culture – Stimulated T-cells are created by combining thawed T-cells with a chemical agent and then labeled with a fluorescent dye before co-culturing with organoids. Unstimulated immune cells serve as negative controls.
Step 8) Automated time-lapse imaging
Automated, live-cell imaging is conducted every few hours using our advanced, high-content solution, ImageXpress® Confocal HT.ai High-Content Imaging System equipped with a high-resolution camera and specialized software to capture 3D structures. These images are then fed into IN Carta Image Analysis Software that uses advanced Artificial Intelligence (AI) to transform images into easily interpretable results.
AI-enabled data analysis – AI-powered IN Carta software can drastically enhance how researchers study T-cell interaction with 3D models. It uses advanced techniques like deep learning-based segmentation and machine learning-based classification to analyze images and categorize data, helping researchers create accurate and efficient assessments of T-cell behavior.
In this example, researchers use IN Carta during the deep learning segmentation to easily generate masks for organoids and the machine learning classification phase to classify organoids into intact organoids (Pink) and modified organoids (Green), which shows an increased number of modified organoids over time.
T-cell applications and assays for high-content screening
Cutting-edge 3D imaging and data analysis methods are crucial in carrying out T-cell and organoid co-culture research for immunotherapy. Such workflows can benefit greatly from high-content imaging, automation, and AI-enabled data analysis software. Learn more about our methods for T-cell assays from the resources below.